We strive to generate and apply polymer materials, both via synthetic and biological methodologies, equipped with both, dynamic and adaptive properties. Focus areas are the molecular design of functional polymers (synthesis, novel functionalization strategies) via control of inter/intramolecular interactions. Our generated materials address self-healing polymers, biomimetic diagnostic/delivery systems (artificial membranes, protein/polymer conjugates) and concepts for charge-storage-materials (ionic liquids, batteries, fuel cell membranes).
The research activities of our group have recently been covered by MRS-TV – see the following LINK to watch the video !
https://www.youtube.com/watch?v=SB9GlbRN6R4&list=PLGVe6BxyFHNVt9kAJpMRHE5MpUMZOypF3&index=7
Research fields
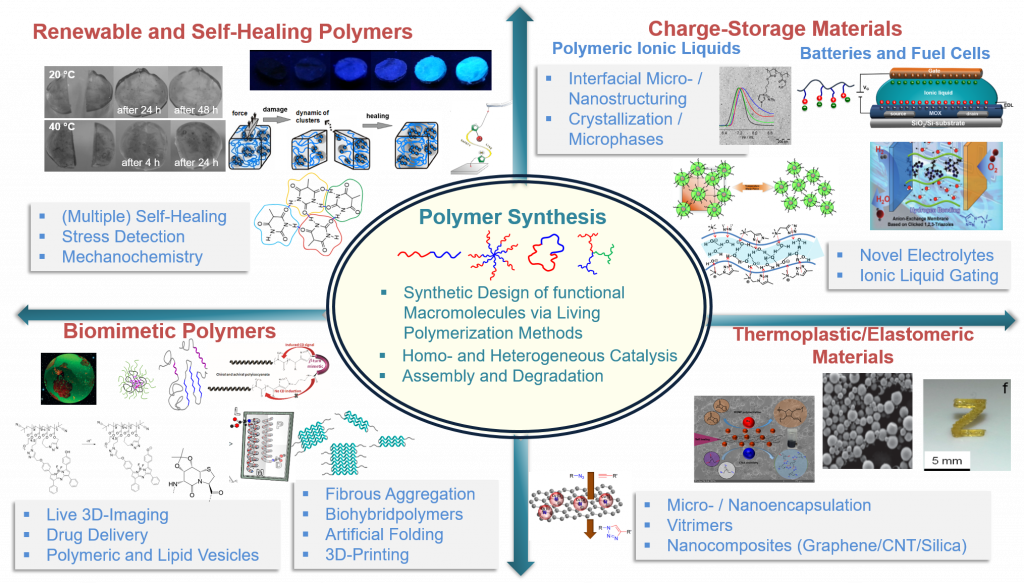
Figure modified from the references 4, 17, 21, 31, 38, 39, 41, 48, 52 with permission including the corresponding copyrights.
- Generate biomimetic, renewable, vitimeric and self-healing polymers 4,23,24-26(research field II)
- Synthesis and novel analytical methods for the preparation of complex macromolecular architectures9,10,13,27-29 (research field I)
- Design of nanoscaled medical imaging systems(single chain nanoparticles) and drug-delivery systems 1,9,11,18,22,30 (research field V)
- Develop novel electrolytes for battery and transistor systems 5,29,31 (research field IV)
- Transfer self-healing concepts into thermoplastic materials, elastomers and electrode-materials3 7,20,21,25,32-34 (research field II)
- Study biological folding, aggregation- and chirality transfer-principles 19,27,35 (research field III)
- 3D-printing of polymers 8,16,36 (research field V)
Macromolecules are key molecules, indispensable for modern society. They are not only present as widely known structural materials in eg. automobiles or aeroplanes, but are found in biomedicine, modern energy- or information technology37. Research focus in our group is the preparation of functional polymers and their application in modern technology, designing polymers for medicine, as advanced structural materials, or for novel batteries and transistors.
Based on the polymer’s structural complexity we use all known living polymerization (such as ATPR, RAFT, NMP, ROMP, LCCP, LAP and ROP-methodologies)2,9,10,13,14,18,19,21-23,38,39 and modern functionalization strategies known from synthetic organic chemistry, including „click“-based methods40,41. Novel polymeric architectures (functional graft-, cyclic-, star-polymers)42 and the site-specific integration of supramolecular interactions (such as hydrogen bonds, ionomers, mechanophores)43,44 into tailored macromolecules allow to generate advanced materials in the areas of biomedicine2,3,9-11,14,22,45,46, modern imaging technology2,18,22, batteries5,7,47, transistors or self-healing-features26,45,48,49. Using modern high resolution mass spectrometry (LC/ESI-MS and GPC/LC-MALDI-methods) and complex hyphenated technologies (two-dimensional chromatography (2D-LC/GPC)50), advanced functions of macromolecules can be integrated by use of 3D-printing technologies.7,8,16,17,51
References
- Wu, Y. ,et al., Hydrogen-bonded supramolecular polymer micelles with pH/photothermal-responsive carmofur release and combined chemo-photothermal therapy. Polymer Chemistry 2022, 13 (8), DOI:https://doi.org/10.1039/D1PY01634B.
- Thümmler, J. F., et al., Tuning the Internal Compartmentation of Single-Chain Nanoparticles as Fluorescent Contrast Agents. Macromolecular Rapid Communications 2022, n/a (n/a), 2200618, DOI:https://doi.org/10.1002/marc.202200618.
- Paschold, A., et al., Modulating the Fibrillization of Parathyroid-Hormone (PTH) Peptides: Azo-Switches as Reversible and Catalytic Entities. Biomedicines 2022, 10 (7), 1512, DOI:https://doi.org/10.3390/biomedicines10071512.
- Krishnan, B. P., et al., Design, Synthesis and Characterization of Vitrimers with Low Topology Freezing Transition Temperature. Polymers 2022, 14 (12), 2456, DOI:https://doi.org/10.3390/polym14122456. Krishnakumar, B., et al., Vitrimers based on bio-derived chemicals: Overview and future prospects. Chemical Engineering Journal 2022, 433, 133261, DOI:https://doi.org/10.1016/j.cej.2021.133261.
- (a) Katcharava, Z., et al., 3D Printable Composite Polymer Electrolytes: Influence of SiO2 Nanoparticles on 3D-Printability. Nanomaterials 2022, 12 (11), 1859, DOI:https://doi.org/10.3390/nano12111859. (b) Li, C., et al., Synthesis and Characterization of Quadrupolar-Hydrogen-Bonded Polymeric Ionic liquids for Potential Self-Healing Electolytes. Polymers, 2022, 14 (19), 4090, DOI:https://doi.org/10.3390/polym14194090.
- Hilgeroth, P. S., et al., 3D Printing of Triamcinolone Acetonide in Triblock Copolymers of Styrene-Isobutylene-Styrene as a Slow-Release System. Polymers 2022, 14 (18), 3742, DOI:https://doi.org/10.3390/polym14183742. Shinde, K. S., et al., A mechanochemically active metal-organic framework (MOF) based on Cu-bis-NHC-linkers: synthesis and mechano-catalytic activation. Macromolecular Chemistry and Physics 2022, 2200207, DOI:https://doi.org/10.1002/macp.202200207.
- Rupp, H., et al., Printable Electrolytes: tuning 3D printing by multiple hydrogen bonds and added inorganic lithium-salts (LiTFSI). Advanced Materials Technology 2022, 7, 2200088, DOI:https://doi.org/10.1002/admt.202200088.
- Du, F., et al., 3D-printing of the polymer/insect-repellent system poly(l-lactic acid)/ethyl butylacetylaminopropionate (PLLA/IR3535). International Journal of Pharmaceutics 2022, 624, 122023, DOI:https://doi.org/10.1016/j.ijpharm.2022.122023.
- Chen, S., et al., Hydrogen-Bonds-Mediated Nanomedicine: Design, Synthesis, and Applications. Macromolecular Rapid Communications 2022, 2200168, DOI:https://doi.org/10.1002/marc.202200168.
- Chen, S., et al., Hydrogen-Bonded Supramolecular Polymer Adhesives: Straightforward Synthesis and Strong Substrate Interaction. Angewandte Chemie International Edition 2022, 61, e202203876, DOI:https://doi.org/10.1002/anie.202203876.
- Cai, Y., et al., Bergman cyclization of main-chain enediyne polymers for enhanced DNA cleavage. Polymer Chemistry 2022, 13 (23), 3412-3421, DOI:https://doi.org/10.1039/D2PY00259K.
- Binder, W. H., et al., Kunststofftechnik: Thermischen Abbau erkennen. Nachrichten aus der Chemie 2022, 70 (1), 40-41, DOI:https://doi.org/10.1002/nadc.20224120686.
- Zheng, X., et al., Halogen-Bond Mediated 3D Confined Assembly of AB Diblock Copolymer and C Homopolymer Blends. Small 2021, 2007570, DOI:https://doi.org/10.1002/smll.202007570.
- Sen, N., et al., Membrane Anchored Polymers Modulate Amyloid Fibrillation. Macromolecular Rapid Communications 2021, 2100120, DOI:https://doi.org/10.1002/marc.202100120.
- Sammon, M. S., et al., Activation of a Copper Biscarbene Mechano-Catalyst Using Single-Molecule Force Spectroscopy Supported by Quantum Chemical Calculations. Chemistry – A European Journal 2021, 27 (34), 8723-8729, DOI:https://doi.org/10.1002/chem.202100555. Raimondo, M., et al., Atomic Force Microscopy Analysis of Supramolecular Self-Responsive Nanocomposites. Polymers 2021, 13 (9), DOI:https://doi.org/10.3390/polym13091401. Mordvinkin, A., et al., Rheology, Sticky Chain, and Sticker Dynamics of Supramolecular Elastomers Based on Cluster-Forming Telechelic Linear and Star Polymers. Macromolecules 2021, 54 (11), 5065-5076, DOI:https://doi.org/10.1021/acs.macromol.1c00655. Guadagno, L., et al., Functional structural nanocomposites with integrated self-healing ability. Materials Today: Proceedings 2021, 34, 243-249. DOI:https://doi.org/10.1016/j.matpr.2020.03.051. Funtan, A., et al., Selfdiagnostic Polymers – Inline Detection of Thermal Degradation of Unsaturated Poly(ester imide)s. Advanced Materials 2021, DOI:https://doi.org/10.1002/adma.202100068.Chen, S., et al., Synthesis and self-aggregated nanostructures of hydrogen-bonding polydimethylsiloxane. Polymer Chemistry 2021, 12 (28), 4111-4119, DOI: https://doi.org/10.1039/D1PY00513H. AG, A.; Funtan, A. Detection of degradation products of a polymer or polymer composition. 2021.upp, H., et al., 3D Printing of Solvent-Free Supramolecular Polymers. Frontiers in Chemistry 2021, 9, 771974, DOI:https://doi.org/10.3389/fchem.2021.771974.
- Rupp, H., et al., Multicomponent Stress-Sensing Composites Fabricated by 3D-Printing Methodologies. Macromolecular Rapid Communications 2021, 2000450, DOI:https://doi.org/10.1002/marc.202000450.
- Rupp, H., et al., Multicomponent Stress-Sensing Composites Fabricated by 3D-Printing Methodologies. Macromolecular Rapid Communications 2021, 2000450, DOI:https://doi.org/10.1002/marc.202000450.
- Roos, A. H., et al., Nanoscale structure and dynamics of thermoresponsive single-chain nanoparticles investigated by EPR spectroscopy. Soft Matter 2021, 17 (29), 7032-7037, DOI:https://doi.org/10.1039/D1SM00582K.
- Rohmer, M., et al., Chiral amines as initiators for ROP and their chiral induction on poly(2-aminoisobutyric acid) chains. Polymer Chemistry 2021, 12 (43), 6252-6262, DOI: https://doi.org/10.1039/D1PY01021B.
- Marinow, A., et al., Materialien für die “Ewigkeit”: selbstheilende Polymere – auch rezyklierbar! Chemie in unserer Zeit 2021, 55(6), 422-433 . DOI:https://doi.org/10.1002/ciuz.202100014.
- Li, C., et al., Comparing C2=O and C2=S Barbiturates: Different Hydrogen-Bonding Patterns of Thiobarbiturates in Solution and the Solid State. International Journal of Molecular Sciences 2021, 22 (23), 12679, DOI:https://doi.org/10.3390/ijms222312679.
- Hoffmann, J. F., et al., Fluorescent and Water Dispersible Single-Chain Nanoparticles: Core–Shell Structured Compartmentation. Angewandte Chemie International Edition 2021, 60 (14), 7820-7827, DOI:https://doi.org/10.1002/anie.202015179.
- Adjedje, V. K. B., et al., Enzymatic degradation of synthetic polyisoprenes via surfactant-free polymer emulsification. Green Chemistry 2021, 23 (23), 9433-9438, DOI:https://doi.org/10.1039/D1GC03515K.
- Chen, S., et al., Opposing Phase-Segregation and Hydrogen-Bonding Forces in Supramolecular Polymers. Angewandte Chemie International Edition 2017, 56 (42), 13016-13020, DOI:http://dx.doi.org/10.1002/anie.201707363. Chen, S., et al., Self-Healing Materials from V- and H-Shaped Supramolecular Architectures. Angew. Chem., Int. Ed. 2015, 54 (35), 10188-10192, DOI:http://dx.doi.org/10.1002/anie.201504136.
- Krishnakumar, B., et al., Catalyst free self-healable vitrimer/graphene oxide nanocomposites. Composites Part B: Engineering 2020, 184, 107647, DOI:https://doi.org/10.1016/j.compositesb.2019.107647.
- Krishnakumar, B., et al., Vitrimers: Associative dynamic covalent adaptive networks in thermoset polymers. Chemical Engineering Journal 2020, 385, 123820, DOI:https://doi.org/10.1016/j.cej.2019.123820.
- Freudenberg, J., et al., Chirality Control of Screw-Sense in Aib-Polymers: Synthesis and Helicity of Amino Acid Functionalized Polymers. ACS Macro Letters 2020, 686-692, DOI:https://doi.org/10.1021/acsmacrolett.0c00218.
- Freudenberg, J., et al., Multisegmented Hybrid Polymer Based on Oligo-Amino Acids: Synthesis and Secondary Structure in Solution and in the Solid State. Macromolecules 2019, 52 (12), 4534-4544, DOI:https://doi.org/10.1021/acs.macromol.9b00684. Biewend, M., et al., Synthesis of polymer-linked copper(i) bis(N-heterocyclic carbene) complexes of linear and chain extended architecture. Polymer Chemistry 2019, 10 (9), 1078-1088, DOI:http://dx.doi.org/10.1039/C8PY01751D. Narumi, A., et al., Evaluation of Ring Expansion-Controlled Radical Polymerization System by AFM Observation. ACS Macro Letters 2019, 8 (6), 634-638, DOI:https://doi.org/10.1021/acsmacrolett.9b00308.
- Chen, S., et al., Synthesis and Morphology of Semifluorinated Polymeric Ionic Liquids. Macromolecules 2018, 51 (21), 8620-8628, DOI:https://doi.org/10.1021/acs.macromol.8b01624.
- Kumar, S., et al., Thio-Bromo “Click” Reaction Derived Polymer–Peptide Conjugates for Their Self-Assembled Fibrillar Nanostructures. Macromolecular Bioscience 2020, 2000048, DOI:https://doi.org/10.1002/mabi.202000048. Funtan, S., et al., Biomimetic Elastin-Like Polypeptides as Materials for the Activation of Mechanophoric Catalysts. Organic Materials 2020, 02 (02), 116-128, DOI:https://doi.org/10.1055/s-0040-1702149. Kumar, S., et al., One-Pot Synthesis of Thermoresponsive Amyloidogenic Peptide–Polymer Conjugates via Thio–Bromo “Click” Reaction of RAFT Polymers. Macromolecular Rapid Communications 2017, 1700507, DOI:http://dx.doi.org/10.1002/marc.201700507. Thümmler, J. F., et al., Tuning the Internal Compartmentation of Single-Chain Nanoparticles as Fluorescent Contrast Agents. Macromolecular Rapid Communications, 2022, 2200618, DOI:https://doi.org/10.1002/marc.202200618.
- Chen, S., et al., Gating effects of conductive polymeric ionic liquids. Journal of Materials Chemistry C 2018, 6 (30), 8242-8250, DOI:https://doi.org/10.1039/C8TC01936C.
- Raimondo, M., et al., Multifunctionality of structural nanohybrids: the crucial role of carbon nanotube covalent and non-covalent functionalization in enabling high thermal, mechanical and self-healing performance. Nanotechnology 2020, 31 (22), 225708, DOI:https://doi.org/10.1088/1361-6528/ab7678.
- Guadagno, L., et al., Functional structural nanocomposites with integrated self-healing ability. Materials Today: Proceedings , 2021, 34(1) Pages 243-249, DOI:https://doi.org/10.1016/j.matpr.2020.03.051. Biewend, M., et al., Detection of stress in polymers: mechanochemical activation of CuAAC click reactions in poly(urethane) networks. Soft Matter 2020, 16 (5), 1137-1141, DOI:https://doi.org/10.1039/C9SM02185J. Michael, P., et al., Mechanochemical Activation of Fluorogenic CuAAC “Click” Reactions for Stress-Sensing Applications. Macromolecular Rapid Communications,2018, 39 (22), 1800376, DOI: https://doi.org/10.1002/marc.201800376.
- Binder, W. H. „Honig“ bis „Gummi“: Die variablen Eigenschaften des Polyisobutylen. 100 Jahre Makromolekulare Chemie. . In Faszination Chemie, die Informationsplattform der GDCh., GDCh: 2020.
- Evgrafova, Z., et al., Modulation of amyloid β peptide aggregation by hydrophilic polymers. Physical Chemistry Chemical Physics 2019, 21 (37), 20999-21006, DOI:https://doi.org/10.1039/C9CP02683E. Evgrafova, Z., et al., Probing Polymer Chain Conformation and Fibril Formation of Peptide Conjugates. ChemPhysChem 2019, 20 (2), 236-240, DOI:https://doi.org/10.1002/cphc.201800867. Deike, S., et al., Induction of Chirality in β-Turn Mimetic Polymer Conjugates via Postpolymerization “Click” Coupling. Macromolecules 2017, 50 (7), 2637-2644, DOI:https://doi.org/10.1021/acs.macromol.7b00343.
- Rupp, H., et al., 3D Printing of Supramolecular Polymers: Impact of Nanoparticles and Phase Separation on Printability. Macromolecular Rapid Communications 2019, 1900467, DOI:https://doi.org/10.1002/marc.201900467.
- Binder, W. H. The Past 40 Years of Macromolecular Sciences: Reflections on Challenges in Synthetic Polymer and Material Science. Macromolecular Rapid Communications 2019, 40 (1), 1800610, DOI:https://doi.org/10.1002/marc.201800610.
- Kurzhals, S., et al., Combination of Olefin Metathesis Polymerization with Click Chemistry. In Handbook of Metathesis, Edition: 2nd, Robert H. Grubbs, E. K. Ed.; John Wiley & Sons, 2015; pp 207-227, DOI:https://doi.org/10.1002/9783527674107.ch35. Binder, W. H., et al., ‘Click’ chemistry in macromolecular synthesis. In Encyclopedia of Polymer Science and Technology, Vol. 3; John Wiley & Sons, Inc., 2014, Vol.3, pp 186-230, DOI:https://doi.org/10.1002/0471440264.pst565. Binder, W. H., et al., Click chemistry in polymer science. In McGraw-Hill Yearbook of Science & Technology, Blumel, D. Ed.; McGraw-Hill, 2011; pp 46-49.
- Kumar, S., et al., Thio-Bromo “Click” Reaction Derived Polymer–Peptide Conjugates for Their Self-Assembled Fibrillar Nanostructures. Macromolecular Bioscience 2020, 20 (6), 2000048, DOI:https://doi.org/10.1002/mabi.202000048.
- Neumann, S., et al., The CuAAC: Principles, Homogeneous and Heterogeneous Catalysts, and Novel Developments and Applications. Macromolecular Rapid Communications 2019, 1900359, DOI:https://doi.org/10.1002/marc.201900359. Li, N., et al., Click-Chemistry for Nanoparticle-Modification. J. Mater. Chem. 2011, 21 (42), 16717 – 16734, DOI:https://doi.org/10.1039/C1JM11558H. Binder, W. H., et al., “Click”-Chemistry in Polymer and Material Science: An Update. Macromol. Rapid Commun. 2008, 29, 952-981, DOI:http://dx.doi.org/10.1002/marc.200800089. Binder, W. H.,et al., “Click” Chemistry in Polymer and Materials Science. Macromol. Rapid Commun. 2007, 28 (1), 15-54. Binder, W. H., et al., Azide/alkyne-“click” reactions: applications in material science and organic synthesis. Curr. Org. Chem. 2006, 10 (14), 1791-1815, DOI:https://doi.org/10.2174/138527206778249838.
- Siva Prasanna Sanka, R. V., et al., Nitrogen-doped graphene stabilized copper nanoparticles for Huisgen [3+2] cycloaddition “click” chemistry. Chemical Communications 2019, 55 (44), 6249-6252, DOI:https://doi.org/10.1039/C9CC02057H.
- Shaygan Nia, A., et al., Graphene as initiator/catalyst in polymerization chemistry. Progress in Polymer Science 2017, 67, 48-76, DOI:https://doi.org/10.1016/j.ijheh.2013.07.004.1016/j. Haryono, A., et al., Controlled Arrangement of Nanoparticle Arrays in Block-Copolymer Domains. Small 2006, 2 (5), 600-611, DOI:http://dx.doi.org/10.1002/smll.200500474. Binder, W. H. Supramolecular Assembly of Nanoparticles at Liquid-Liquid Interfaces. Angew. Chem., Int. Ed. 2005, 44 (33), 5172-5175, DOI:https://doi.org/10.1002/anie.200501220.
- Enke, M., et al., Intrinsic Self-Healing Polymers Based on Supramolecular Interactions: State of the Art and Future Directions. Adv. Polym. Sci. 2016, 59-112, DOI:http://dx.doi.org/10.1007/12_2015_345. Chen, S., et al., Dynamic Ordering and Phase Segregation in Hydrogen-Bonded Polymers. Acc. Chem. Res. 2016, 49 (7), 1409-1420, DOI:http://dx.doi.org/10.1021/acs.accounts.6b00174. Binder, W., et al., Supramolecular Polymers and Networks with Hydrogen Bonds in the Main- and Side-Chain. In Adv. Polym. Sci.: “Hydrogen Bonded Polymers”, Binder, W. H. Ed.; Advances in Polymer Science, 2007; pp 1-78 , DOI:http://dx.doi.org/10.1007/12_2006_109.
- Li, N., et al., Towards High Conductivity in Anion-Exchange Membranes for Alkaline Fuel Cells. ChemSusChem 2013, 6 (8), 1376–1383, DOI:https://doi.org/10.1002/cssc.201300320. Stojanovic, A., et al., Designing melt flow of poly(isobutylene)-based ionic liquids. J. Mater. Chem. A 2013, 1 (39), 12159-12169, DOI:http://dx.doi.org/10.1039/C3TA12646C.
- Döhler, D., et al., Biomimetische Materialien: Selbstheilende Polymere. Chemie in unserer Zeit 2016, 50 (2), 90-101, DOI:http://dx.doi.org/10.1002/ciuz.201500686.
- Schulz, M., et al., Mixed Hybrid Lipid/Polymer Vesicles as a Novel Membrane Platform. Macromol. Rapid Commun. 2015, 36 (23), 2031-2041, DOI:http://dx.doi.org/10.1002/marc.201500344. Binder, W. H. Polymer-Induced Transient Pores in Lipid Membranes. Angew. Chem., Int. Ed. 2008, 47 (17), 3092-3095, DOI:http://dx.doi.org/10.1002/anie.200800269. Binder, W. H., et al., Self-Assembly of Fibers and Fibrils. Angew. Chem., Int. Ed. 2006, 45 (44), 7324-7328, DOI:http://dx.doi.org/10.1002/anie.200602001. Binder, W. H., et al., Domains and Rafts in Lipid Membranes. Angew. Chem., Int. Ed. 2003, 42 (47), 5802-5827, DOI:http://dx.doi.org/10.1002/anie.200300586.
- Frenzel, F., et al., Glassy Dynamics and Charge Transport in Polymeric Ionic Liquids. In Dielectric Properties of Ionic Liquids, Paluch, M., Kremer, F. Eds.; Springer International Publishing Switzerland 2016; pp 115-129, DOI:http://www.springer.com/de/book/9783319324876.
- Campanella, A., et al., Self-Healing in Supramolecular Polymers. Macromolecular Rapid Communications 2018, 1700739, DOI:http://dx.doi.org/10.1002/marc.201700739. Döhler, D., et al., CuAAC-Based Click Chemistry in Self-Healing Polymers. Accounts of Chemical Research 2017, 50 (10), 2610-2620, DOI:http://dx.doi.org/10.1021/acs.accounts.7b00371. Herbst, F., et al., Self-healing polymers via supramolecular forces. Macromol. Rapid Commun. 2013, 34 (3), 203-220, DOI:http://dx.doi.org/10.1002/marc.201200675.
- Herbst, F., et al., Dynamic supramolecular poly(isobutylene)s for self-healing materials. Polymer Chemistry 2012, 3 (11), 3084-3092, DOI:http://dx.doi.org/10.1039/C2PY20265D.
- Barqawi, H., et al., 2D-LC/SEC-(MALDI-TOF)-MS Characterization of Symmetric and Nonsymmetric Biocompatible PEOm–PIB–PEOn Block Copolymers. Macromolecules 2013, 46 (19), 7638-7649, DOI:https://doi.org/10.1021/ma401604h. Barqawi, H., et al., Multidimensional Characterization of α,ω-Telechelic Poly(ε-caprolactone)s via Online Coupling of 2D Chromatographic Methods (LC/SEC) and ESI-TOF/MALDI-TOF-MS. Macromolecules 2012, 45 (24), 9779–9790, DOI:https://doi.org/10.1021/ma3016739. Binder, W. H., et al., Monitoring Block-Copolymer Crossover-Chemistry in ROMP: Catalyst Evaluation via Mass-Spectrometry (MALDI). Macromolecules 2009, 42 (24), 9457-9466, DOI:https://doi.org/10.1021/ma902115j.
- Rupp, H., et al., 3D Printing of Core–Shell Capsule Composites for Post-Reactive and Damage Sensing Applications. Advanced Materials Technologies 2020, 5 (11), 2000509, DOI:https://doi.org/10.1002/admt.202000509. Rupp, H., et al., 3D Printing of Supramolecular Polymers: Impact of Nanoparticles and Phase Separation on Printability. Macromolecular Rapid Communications 2019, 40 (24), 1900467, DOI:https://doi.org/10.1002/marc.201900467.
- Binder, W. H., et al., Melamine-formaldehyde resins. In Encyclopedia of Polymer Science and Technology, Vol. 7; John Wiley & Sons, Inc., 2014; pp 727-743, DOI:https://doi.org/10.1002/0471440264.pst498. Herbst, F., et al., Self-healing polymers via supramolecular, hydrogen bonded networks. In Self Healing Polymers: from Principles to Application, Binder, W. H. Ed.; Wiley-VCH Verlag GmbH & Co. KGaA, 2013; pp 275-300, DOI:https://doi.org/10.1002/9783527670185.ch11.
- Binder *, W. H. Opening up new horizons in self-healing materials. EU Research 2019, 20, DOI:http://www.euresearcher.com/14/eu-research-live. Döhler, D., et al., Von der Muschel zum Autolack. Nachrichten aus der Chemie 2016, 64 (9), 836-841 ,DOI:http://dx.doi.org/10.1002/nadc.20164046036. Schulz, M. et al., Beyond the Lipid-Bilayer: Interaction of Polymers and Nanoparticles with Membranes. Soft Matter 2012, 8 (18), 4849–4864, DOI:http://dx.doi.org/10.1039/C2SM06999G. Herbst, F., et al., Ordentlich Dynamisch : Supramolekulare Polymere. Nachrichten aus der Chemie 2010, 734-740, DOI:http://dx.doi.org/10.1002/nadc.201073367. Binder, W. H. Polymeric Ordering by H-bonds. Mimicking Nature by Smart Building Blocks. Monatsh. Chem. / Chemical Monthly 2005, 136 (1), 1-19, DOI:http://dx.doi.org/10.1007/s00706-004-0236-0. Farnik, D., et al., Synthesis and Self Assembly of Hydrogen-Bonded Supramolecular Polymers. Macromol. Symp. 2004, 217 (1), 247-266, DOI:http://dx.doi.org/10.1002/masy.200451320. Binder, W. H. The “labile” chemical bond: A perspective on mechanochemistry in polymers. Polymer 2020, 202, 122639, DOI:https://doi.org/10.1016/j.polymer.2020.122639. Abd-El-Aziz, A. S., et al., The Next 100 Years of Polymer Science. Macromolecular Chemistry and Physics 2020, 221 (16), 2000216, DOI:https://doi.org/10.1002/macp.202000216.
- Döhler, D., et al., Principles of Self Healing Polymers. In Self Healing Polymers: from Principle to Application, Binder, W. H. Ed.; Wiley-VCH Verlag GmbH & Co. KGaA, 2013; pp 7-60, DOI:https://doi.org/10.1002/9783527670185.ch1. Binder, W. H., et al., Biomimetic Principles in Macromolecular Science. In Bioinspiration and Biomimicry in Chemistry, Swiegers, G. Ed.; John Wiley and Sons, 2012; pp 323-366, DOI:https://doi.org/10.1002/9781118310083.ch11. Binder, W. H., et al., Synthesis and Self-Assembly of Hydrogen-Bonded Supramolecular Polymers. In Complex Macromolecular Architectures, John Wiley & Sons (Asia) Pte Ltd, 2011; pp 53-95, DOI:http://dx.doi.org/10.1002/9780470825150.ch3. Binder, W. H., et al., “Click”-Chemistry in Macromolecular Synthesis. In Encyclopedia of Polymer Science and Technology, John Wiley & Sons, Inc, 2009; p DOI:https://doi.org/10.1002/0471440264.pst565. Binder, W. H., et al., “Click”-chemistry on supramolecular materials. In Click Chemistry for Biotechnology and Materials Science, Lahann, J. Ed.; Wiley-Blackwell, 2009; pp 119-175, DOI: https://doi.org/10.1002/9780470748862.ch7. Binder, W. H. Melamine–Formaldehyde Resins. In Encyclopedia of Polymer Science and Technology, July 15, 2004 ed.; 2004; pp 369-385, DOI:https://doi.org/10.1002/0471440264.pst498.