
Reuse and life-cycle-enhancement of polymers is among the most pressing societal needs. We address novel concepts for reusing thermoplastics and thermosets based on vitrimers1, self-healing materials2, 3 and reusable rubbers, funded by the graduate school AGRIPOLY, DFG- and EU-projects. Both, chemical synthesis and enzymatic degradation( of rubber4 ) are studied to generate polymers with improved life-cycle as modern, environmentally friendly materials5.Recent work adds diagnostic reported systems6, 7 , self-healing electrolytes8 and enzymatic degradation of rubber4.
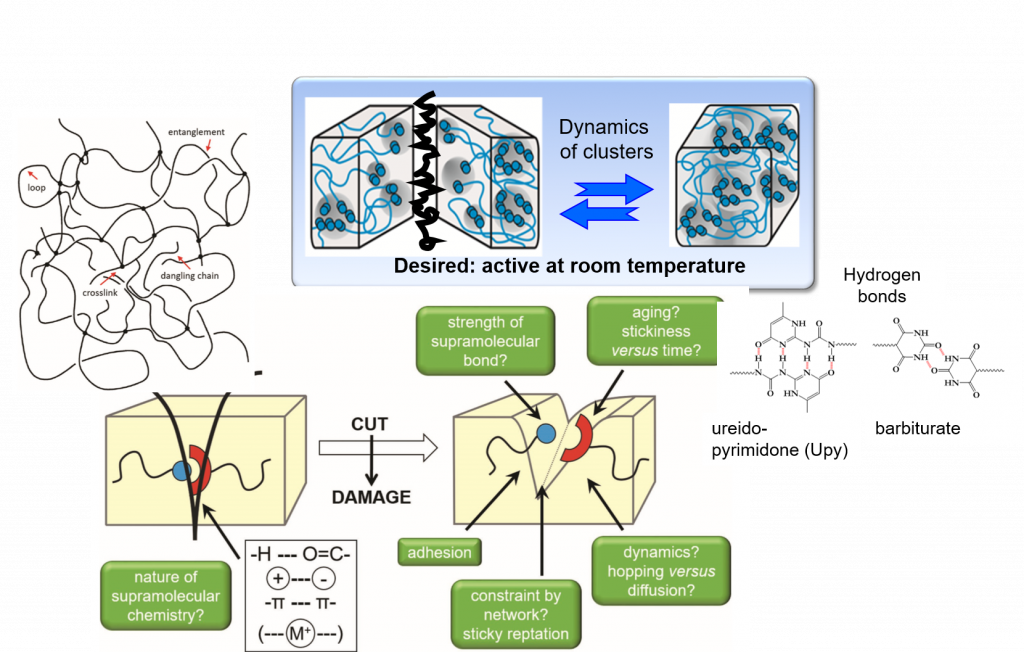
Self-healing is among the great desires in materials, especially if accomplished autonomous and at room temperature conditions – only then an application in everyday life, such as coatings, smartphones, electronics is possible. One focus is placed on the embedding of multiple hydrogen-bonds,9-11 able to introduce self-healing into any desired polymeric materials.2, 12-13 Based on our longstanding tradition in hydrogen-bonds,11 their assembly, their phase-behavior14 and strength in polymers,4, 15-17 we are able to adapt the required strength and properties for the effect needed.12, 18 Proper choice of the hydrogen bond11, 19 in relation to the polymer allows to tune the healing-response, the healing time, and the healing conditions.20-21
Multiple-H-bonds based on barbiturates and complex, chelate-type H-bonds17, 22 in particular are prone to reversible assembly, useful for room-temperature self-healing.18, 19, 23, 24 Different methodologies of synthetic (polymer) chemistry are used to address the incorporation of the H-bonds into the final polymers, such as RAFT, ATRP, NMP, living carbocationic polymerization (LCCP), or living anionic polymerization (LCP). Thermoplastic, elastomeric and also thermoset/composite-materials19, 20, 23-26 are equipped with self-healing properties, the latter using dynamic disulfide-bonds, introducing vitrimeric properties27. We can achieve self-healing at ambient conditions, technologically important when transferred to structural materials and coatings.26, 28 Since in all cases the intrinsic dynamics of the hydrogen bond is important and often different from the solvated state,29 their dynamics9 within the solid material are studied via various physical methodologies, among them melt-rheology,34-36 X-ray scattering,21, 37 or solid state NMR-spectroscopy15 to reveal mechanistic details of such self-healing processes35.We are expanding towards the design of novel H-bonding systems30, self-healing electrolytes8, 31, adhesives32, vitrimers1, 27 and novel drug release systems32, 33.
Our second approach uses encapsulation methods, coupled to a triggered “click”-chemistry to sense and subsequently heal the material.38 Based on the enormous versatility of the CuAAc (copper-catalyzed-click-reaction) as developed by Meldal in 200139-41 and its extreme robustness mainly due to autoacceleration-effects44-45 and chelation-assistance,46 this chemistry can easily be used in stress-induced, catalytic systems. We still work on then improvement of this highly valued self-healing materials using modern multicomponent 3D-printing-technologies42, 45, 47 and its application in nanocomposites26, 43, 48.

Embedding nanosized capsules into the polymers, wherein reactive components are stored, a stress-triggered signal releases the components and activates the catalyst.49 Various self-healing nanocomposites and thermoset-systems, able to heal cracks at ambient temperature within several minutes have been developed.45, 49, 50 Graphene–51 and CNT-based Cu-catalyst48, 52 are specifically attractive for this purpose due to their high stability and excellent dispersibility. With specially designed bis-N-heterocyclic Cu(I)-complexes as mechanophores a direct activation by stress can be achieved,53 allowing to sense and quantify stress inside thermoplastic- and thermoset polymers and biomaterials such as elastin-like peptides.54
Current research activities in this area are using 3D-printed multicomponent materials55 to embed all types of self-healing and self-sensing properties6, 7, 56, 57 in coatings, elastomers, biomaterials, together with the exact quantification of the molecular force required to activate the mechanophores58, 59 via SFMS-methods.
References
- Krishnakumar, B., et al., Vitrimers: Associative dynamic covalent adaptive networks in thermoset polymers. Chemical Engineering Journal 2020, 385, 123820,DOI:https://doi.org/10.1016/j.cej.2019.123820. Krishnan, B. P., et al., Design, Synthesis and Characterization of Vitrimers with Low Topology Freezing Transition Temperature. Polymers 2022, 14 (12), 2456, DOI:https://doi.org/10.3390/polym14122456 Krishnakumar, B., et al., Vitrimers based on bio-derived chemicals: Overview and future prospects. Chemical Engineering Journal 2022, 433, 133261, DOI: https://doi.org/10.1016/j.cej.2021.133261.
- Campanella, A., et al., Self-Healing in Supramolecular Polymers. Macromolecular Rapid Communications 2018, 1700739, DOI:http://dx.doi.org/10.1002/marc.201700739.
- Marinow, A., et al., Materialien für die “Ewigkeit”: selbstheilende Polymere – auch rezyklierbar! Chemie in unserer Zeit, 2021, 55(6), 422-433, DOI: https://doi.org/10.1002/ciuz.202100014.
- Adjedje, V. K. B., et al., Enzymatic degradation of synthetic polyisoprenes via surfactant-free polymer emulsification. Green Chemistry 2021, 23 (23), 9433-9438, DOI:https://doi.org/10.1039/D1GC03515K.
- Binder, W. H., The Past 40 Years of Macromolecular Sciences: Reflections on Challenges in Synthetic Polymer and Material Science. Macromolecular Rapid Communications 2019, 40 (1), 1800610, DOI:https://doi.org/10.1002/marc.201800610.
- Binder, W. H., et al., Kunststofftechnik: Thermischen Abbau erkennen. Nachrichten aus der Chemie 2022, 70 (1), 40-41, DOI:https://doi.org/10.1002/nadc.20224120686.
- Funtan, A., et al., Selfdiagnostic Polymers – Inline Detection of Thermal Degradation of Unsaturated Poly(ester imide)s. Advanced Materials 2021, 33(18)2100068, DOI:https://doi.org/10.1002/adma.202100068.
- Katcharava, Z., et al., 3D Printable Composite Polymer Electrolytes: Influence of SiO2 Nanoparticles on 3D-Printability. Nanomaterials 2022, 12 (11), 1859, DOI:https://doi.org/10.3390/nano12111859.
- Chen, S., et al., Dynamic Ordering and Phase Segregation in Hydrogen-Bonded Polymers. Accounts of Chemical Research 2016, 49 (7), 1409-1420, DOI:http://dx.doi.org/10.1021/acs.accounts.6b00174.
- Herbst, F., et al., Self-healing polymers via supramolecular forces. Macromolecular Rapid Communications 2013, 34 (3), 203-220, DOI:http://dx.doi.org/10.1002/marc.201200675.
- Binder, W., et al., Supramolecular Polymers and Networks with Hydrogen Bonds in the Main- and Side-Chain. In Advanced Polymer Science: “Hydrogen Bonded Polymers”, Binder, W. H., Ed. 2007; pp 1-78, DOI:http://dx.doi.org/10.1007/12_2006_109.
- Herbst, F., et al., Self-healing polymers via supramolecular, hydrogen bonded networks. In Self Healing Polymers: from Principles to Application, Binder, W. H., Ed. Wiley-VCH Verlag GmbH & Co. KGaA: Weinheim, 2013; pp 275-300, DOI:https://doi.org/10.1002/9783527670185.ch11.
- Döhler, D., et al., Principles of Self Healing Polymers. In Self Healing Polymers: from Principle to Application, Binder, W. H., Ed. Wiley-VCH Verlag GmbH & Co. KGaA: Weinheim, 2013; pp 7-60, DOI:https://doi.org/10.1002/9783527670185.ch1. Binder, W. H., et al., Biomimetic Principles in Macromolecular Science. In Bioinspiration and Biomimicry in Chemistry, Swiegers, G., Ed. John Wiley and Sons: Hoboken, New Jersey, 2012; pp 323-366, DOI:https://doi.org/10.1002/9781118310083.ch11.
- Chen, S., et al., Engineering the Morphology of Hydrogen-Bonded Comb-Shaped Supramolecular Polymers: From Solution Self-Assembly to Confined Assembly. Polymer Chemistry 2020, DOI:http://dx.doi.org/10.1039/D0PY00570C.
- Chen, S., et al., Opposing Phase-Segregation and Hydrogen-Bonding Forces in Supramolecular Polymers. Angewandte Chemie International Edition 2017, 56 (42), 13016-13020, DOI:http://dx.doi.org/10.1002/anie.201707363.
- Chen, S., et al., Hierarchical Micelles via Polyphilic Interactions: Hydrogen-Bonded Supramolecular Dendrons and Double Immiscible Polymers. Nano Letters 2016, 16 (2), 1491-1496, DOI:http://dx.doi.org/10.1021/acs.nanolett.5b05203.
- Chen, S., et al., Self-Healing Materials from V- and H-Shaped Supramolecular Architectures. Angewandte Chemie International Edition 2015, 54 (35), 10188-10192, DOI:http://dx.doi.org/10.1002/anie.201504136.
- Binder, W. H., et al., Synthesis and Self-Assembly of Hydrogen-Bonded Supramolecular Polymers. In Complex Macromolecular Architectures, John Wiley & Sons (Asia) Pte Ltd: 2011; pp 53-95, DOI:http://dx.doi.org/10.1002/9780470825150.ch3.
- Enke, M., et al., Intrinsic Self-Healing Polymers Based on Supramolecular Interactions: State of the Art and Future Directions. Advances in Polymer Science 2016, 59-112, DOI:http://dx.doi.org/10.1007/12_2015_345. Döhler, D., et al., Biomimetische Materialien: Selbstheilende Polymere. Chemie in unserer Zeit 2016, 50 (2), 90-101, DOI:http://dx.doi.org/10.1002/ciuz.201500686. Herbst, F., et al., Ordentlich Dynamisch : Supramolekulare Polymere. Nachrichten aus der Chemie 2010, 734-740, DOI:http://dx.doi.org/10.1002/nadc.201073367.
- Herbst, F., et al., Dynamic supramolecular poly(isobutylene)s for self-healing materials. Polymer Chemistry 2012, 3 (11), 3084-3092, DOI:http://dx.doi.org/10.1039/C2PY20265D.
- Herbst, F., et al., Aggregation and chain dynamics in supramolecular polymers by dynamic rheology: cluster formation and self aggregation. Macromolecules 2010, 43, 10006–10016, DOI:http://dx.doi.org/10.1021/ma101962y.
- Hackethal, K., et al., Synthesis and clustering of supramolecular “graft” polymers. Journal of Polymer Science Part A: Polymer Chemistry 2012, 50 (21), 4494-4506, DOI:http://dx.doi.org/10.1002/pola.26257.
- Yan, T., et al., What Controls the Structure and the Linear and Nonlinear Rheological Properties of Dense, Dynamic Supramolecular Polymer Networks? Macromolecules 2017, 50 (7), 2973-2985, DOI:http://dx.doi.org/10.1021/acs.macromol.6b02507.
- Callies, X., et al., Effects of multifunctional cross-linkers on rheology and adhesion of soft nanostructured materials. Soft Matter 2017, 13 (43), 7979-7990, DOI:http://dx.doi.org/10.1039/C7SM01304C.
- Guadagno, L., et al., Functional structural nanocomposites with integrated self-healing ability. Materials Today: Proceedings 2020, DOI:https://doi.org/10.1016/j.matpr.2020.03.051.
- Guadagno, L., et al., Reversible Self-Healing Carbon-Based Nanocomposites for Structural Applications. Polymers 2019, 11 (5), 903, DOI:http://www.mdpi.com/2073-4360/11/5/903. Guadagno, L., et al., Self-healing epoxy nanocomposites via reversible hydrogen bonding. Composites Part B: Engineering 2019, 157, 1-13, DOI:https://doi.org/10.1016/j.compositesb.2018.08.082.
- Krishnakumar, B., et al., Catalyst free self-healable vitrimer/graphene oxide nanocomposites. Composites Part B: Engineering 2020, 184, 107647, DOI:https://doi.org/10.1016/j.compositesb.2019.107647.
- Guadagno, L., et al., Development of self-healing multifunctional materials. Composites Part B: Engineering 2017, 128, 30-38, DOI:https://doi.org/10.1016/j.compositesb.2017.07.003.
- Herbst, F., et al., Comparing solution and melt-state association of hydrogen bonds in supramolecular polymers. Polymer Chemistry 2013, 4, 3602-3609, DOI:http://xlink.rsc.org/?doi=C3PY00362K.
- Li, C., et al., Comparing C2=O and C2=S Barbiturates: Different Hydrogen-Bonding Patterns of Thiobarbiturates in Solution and the Solid State. International Journal of Molecular Sciences 2021, 22 (23), 12679, DOI:https://doi.org/10.3390/ijms222312679.
- Rupp, H., et al., Printable Electrolytes: tuning 3D printing by multiple hydrogen bonds and added inorganic lithium-salts (LiTFSI). Advanced Materials Technology 2022, 7(8), 2200088, DOI:https://doi.org/10.1002/admt.202200088.
- Chen, S.; Wu, Y.; Lortie, F.; Bernard, J.; Binder, W. H.; Zhu, J. Hydrogen-Bonds-Mediated Nanomedicine: Design, Synthesis, and Applications. Macromolecular Rapid Communications 2022, 2200168, DOI:https://doi.org/10.1002/marc.202200168.
- Wu, Y.; Wang, H.; Liu, Q.; Lortie, F.; Bernard, J.; Binder, W. H.; Chen, S.; Zhu, J. Hydrogen-bonded supramolecular polymer micelles with pH/photothermal-responsive carmofur release and combined chemo-photothermal therapy. Polymer Chemistry 2022, 13 (8), 1010-1014, DOI:https://pubs.rsc.org/en/content/articlelanding/2022/py/d1py01634b.
- Chen, S., et al., Rheology of hydrogen-bonded dendritic supramolecular polymer networks in the melt state. Polymer 2016, 107, 466-473, DOI:http://dx.doi.org/10.1016/j.polymer.2016.08.046.
- Yan, T., et al., Unveiling the molecular mechanism of self-healing in a telechelic, supramolecular polymer network. Scientific Reports 2016, 6, 32356, DOI:https://doi.org/10.1038/srep32356.
- Yan, T., et al., Nanostructure and Rheology of Hydrogen-Bonding Telechelic Polymers in the Melt: From Micellar Liquids and Solids to Supramolecular Gels. Macromolecules 2014, 47 (6), 2122-2130, DOI:http://dx.doi.org/10.1021/ma402007f.
- Ostas, E., et al., Crystallization of Supramolecular Pseudoblock Copolymers. Macromolecules 2013, 46 (11), 4481-4490, DOI:http://dx.doi.org/10.1021/ma400622w. Ostas, E., et al., Poly(ε-caprolactone)–poly(isobutylene): A crystallizing, hydrogen-bonded pseudo-block copolymer. Journal of Polymer Science Part A: Polymer Chemistry 2011, 49 (15), 3404-3416, DOI:http://dx.doi.org/10.1002/pola.24777.
- Döhler, D., et al., CuAAC-Based Click Chemistry in Self-Healing Polymers. Accounts of Chemical Research 2017, 50 (10), 2610-2620, DOI:http://dx.doi.org/10.1021/acs.accounts.7b00371.
- Neumann, S., et al., The CuAAC: Principles, Homogeneous and Heterogeneous Catalysts, and Novel Developments and Applications. Macromolecular Rapid Communications 2019, 1900359, DOI:https://doi.org/10.1002/marc.201900359.
- Binder, W. H., et al., “Click”-Chemistry in Polymer and Material Science: An Update. Macromolecular Rapid Communications 2008, 29, 952-981, DOI:http://dx.doi.org/10.1002/marc.200800089.
- Binder, W. H., et al., “Click” Chemistry in Polymer and Materials Science. Macromolecular Rapid Communications 2007, 28 (1), 15-54, DOI:https://doi.org/10.1002/marc.200600625.
- Rupp, H., et al., Multicomponent Stress-Sensing Composites Fabricated by 3D-Printing Methodologies. Macromolecular Rapid Communications 2021, 2000450, DOI:https://doi.org/10.1002/marc.202000450. Rupp, H., et al., 3D Printing of Core–Shell Capsule Composites for Post-Reactive and Damage Sensing Applications. Advanced Materials Technologies 2020, 2000509, DOI:https://doi.org/10.1002/admt.202000509.
- Raimondo, M., et al., Tunneling Atomic Force Microscopy Analysis of Supramolecular Self-Responsive Nanocomposites. Polymers 2021, 13 (9), 1401, DOI:https://www.mdpi.com/2073-4360/13/9/1401. Guadagno, L., et al., Functional structural nanocomposites with integrated self-healing ability. Materials Today: Proceedings 2021, 34, 243-249, DOI:https://www.sciencedirect.com/science/article/pii/S2214785320318253.
- Döhler, D., et al., Autocatalysis in the Room Temperature Copper(I)-Catalyzed Alkyne–Azide “Click” Cycloaddition of Multivalent Poly(acrylate)s and Poly(isobutylene)s. Macromolecules 2012, 45 (8), 3335-3345, DOI:http://dx.doi.org/10.1021/ma300405v.
- Kargarfard, N., et al., Improving Kinetics of “Click-Crosslinking” for Self-Healing Nanocomposites by Graphene-Supported Cu-Nanoparticles. Polymers 2018, 10 (1), 17, DOI: https://doi.org/10.3390/polym10010017.
- Neumann, S., et al., Chelation-assisted CuAAC in star-shaped polymers enables fast self-healing at low temperatures. Polymer Chemistry 2016, 7 (13), 2342-2351, DOI:http://dx.doi.org/10.1039/C5PY01818H.
- Rupp, H., et al., 3D Printing of Solvent-Free Supramolecular Polymers. Frontiers in Chemistry 2021, 9, 771974, DOI:https://doi.org/10.3389/fchem.2021.771974.
- Siva Prasanna Sanka, R. V., et al., Nitrogen-doped graphene stabilized copper nanoparticles for Huisgen [3+2] cycloaddition “click” chemistry. Chemical Communications 2019, 55 (44), 6249-6252, DOI:http://dx.doi.org/10.1039/C9CC02057H.
- Schunack, M., et al., Low-Temperature Cu(I)-Catalyzed “Click” Reactions for Self-Healing Polymers. Macromolecular Chemistry and Physics 2012, 213 (2), 205-214, DOI:http://dx.doi.org/10.1002/macp.201100377. Gragert, M., et al., Azide/Alkyne-“Click”-Reactions of Encapsulated Reagents: Toward Self-Healing Materials. Macromolecular Rapid Communications 2011, 32 (5), 419-425, DOI:http://dx.doi.org/10.1002/marc.201000687.
- Rana, S., et al., “Click”-Triggered Self-Healing Graphene Nanocomposites. Macromolecular Rapid Communications 2016, 37 (21), 1715-1722, DOI:http://dx.doi.org/10.1002/marc.201600466. Shaygan Nia, A., et al., Nanocomposites via a direct graphene-promoted “click”-reaction. Polymer 2015, 79, 21-28, DOI:http://dx.doi.org/10.1016/j.polymer.2015.10.013. Vasiliu, S., et al., Insights into the Mechanism of Polymer Coating Self-Healing Using Raman Spectroscopy. Applied Spectroscopy 2014, 68 (5), 541-548, DOI:http://as.osa.org/abstract.cfm?URI=as-68-5-541. Guadagno, L., et al., Evaluation of the Mechanical Properties of Microcapsule-Based Self-Healing Composites. International Journal of Aerospace Engineering 2016, 10, DOI:http://dx.doi.org/10.1155/2016/7817962.
- Shaygan Nia, A., et al., Graphene as initiator/catalyst in polymerization chemistry. Progress in Polymer Science 2017, 67, 48-76, DOI:http://dx.doi.org/10.1016/j.progpolymsci.2016.12.005.
- Shaygan Nia, A., et al., Carbon-Supported Copper Nanomaterials: Recyclable Catalysts for Huisgen [3+2] Cycloaddition Reactions. Chemistry – A European Journal 2015, 21 (30), 10763-10770, DOI:http://dx.doi.org/10.1002/chem.201501217. Shaygan Nia, A., et al., Click chemistry promoted by graphene supported copper nanomaterials. Chemical Communications 2014, 50 (97), 15374-15377, DOI:http://dx.doi.org/10.1039/C4CC07774A.
- Biewend, M., et al., Detection of stress in polymers: mechanochemical activation of CuAAC click reactions in poly(urethane) networks. Soft Matter 2020, 16 (5), 1137-1141, DOI:http://dx.doi.org/10.1039/C9SM02185J. Biewend, M., et al., Synthesis of polymer-linked copper(i) bis(N-heterocyclic carbene) complexes of linear and chain extended architecture. Polymer Chemistry 2019, 10 (9), 1078-1088, DOI:http://dx.doi.org/10.1039/C8PY01751D. Michael, P., et al., Synthesis and characterization of polymer linked copper(I) bis(N-heterocyclic carbene) mechanocatalysts. Journal of Polymer Science Part A: Polymer Chemistry 2017, 55 (23), 3893-3907, DOI:http://dx.doi.org/10.1002/pola.28775. Michael, P., et al., A Mechanochemically Triggered “Click” Catalyst. Angewandte Chemie International Edition 2015, 54 (47), 13918-13922, DOI:http://dx.doi.org/10.1002/anie.201505678.
- Funtan, S., et al., Biomimetic Elastin-Like Polypeptides as Materials for the Activation of Mechanophoric Catalysts. Organic Materials 2020, 2 (2), 116-128, DOI:https://doi.org/10.1055/s-0040-1702149. Funtan, S., et al., Synthesis and Mechanochemical Activity of Peptide-Based Cu(I) Bis(N-Heterocyclic Carbene) Complexes. Biomimetics 2019, 4 (1), 24, DOI:https://doi.org/10.3390/biomimetics4010024.
- Rupp, H., et al., 3D Printing of Supramolecular Polymers: Impact of Nanoparticles and Phase Separation on Printability. Macromolecular Rapid Communications 2019, 1900467, DOI:https://doi.org/10.1002/marc.201900467.
- Döhler, D., et al., Qualitative sensing of mechanical damage by a fluorogenic “click” reaction. Chemical Communications 2016, 52 (74), 11076-11079, DOI:http://dx.doi.org/10.1039/C6CC05390D.
- Hu, M., et al., Monitoring crack appearance and healing in coatings with damage self-reporting nanocapsules. Materials Horizons 2018, 5 (1), 51-58, DOI:http://dx.doi.org/10.1039/C7MH00676D.
- Binder, W. H., The “labile” chemical bond: A perspective on mechanochemistry in polymers. Polymer 2020, 122639, DOI:https://doi.org/10.1016/j.polymer.2020.122639.
- Michael, P., et al., Mechanochemical Activation of Fluorogenic CuAAC “Click” Reactions for Stress-Sensing Applications. Macromolecular Rapid Communications 2018, 1800376, DOI:https://doi.org/10.1002/marc.201800376.